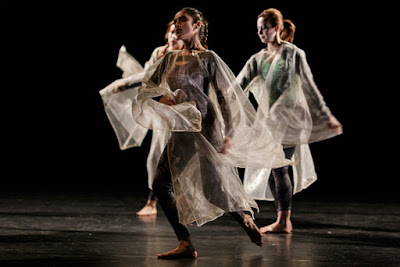 |
Image source: "Dunamis Novem" link below |
Topics: NIST, Quantum Mechanics, Research, STEAM
Quantum physics drives much of the research at the National Institute of Standards and Technology (NIST). Explaining this research is a challenge, because quantum physics—nature's rules for the smallest particles of matter and light—inspires words like weird, curious, and counter-intuitive. The quantum world is strange and invisible in the context of everyday life. And yet, quantum physics can be explained and at least partially demonstrated visually.
NIST physicist Ray Simmonds recently collaborated with MFA graduate candidate Sam Mitchell of the University of California, San Diego (UCSD), to create a dance piece based on the laws of quantum physics. The piece, Dunamis Novem (Latin for "the chance happening of nine things"),* premiered at The La Jolla Playhouse Forum Theatre in January, as a part of Mitchell's thesis work.
The project has practical benefits such as education, Simmonds says.
"While quantum mechanics is a well-established theory, proven true overwhelmingly by experiments, it is still confounding to most people, even those in science," Simmonds and Mitchell noted in describing their work.
“Quantum Statistics: Affects on Human Dancers and the Observer”
Abstract
The Arts and Sciences may seem to be immiscible fields of study, even at odds with each other. In Leonardo Da Vinci’s time these two fields were not polarized, in fact, they coexisted naturally. Despite the appearance of being far distant cousins, both artists and scientists share a creative gene, a passion for their work, and a brave curiosity that pushes them past current boundaries to explore the unknown. In this lecture, we will present some recent examples of those mixing these two worlds and our own attempts to do so with Dance Theater and Quantum Physics. While quantum mechanics is a well-established theory, proven true overwhelmingly by experiments, it is still confounding to most people, even those in science. At its heart, it describes nature in terms of possible realities with probable outcomes, with almost no predictable certainty. Experts still struggle to interpret its philosophical consequences and the notion that there may be no “objective reality”. Even Albert Einstein, one of its co-creators, disapproved of its bizarre properties, saying that “God does not play dice with the universe”. In the creation of this work, “Dunamis Novem”, we have taken some of the probabilistic rules that govern quantum systems and integrated them into a creative process. The results are then born from an artistic aesthetic and an algorithmic code that produces dynamics that embody in some way randomness, concepts of “quantum entanglement”, and the effects of observation or “measurement”. Our work shows that “Science” can inspire and direct new forms of “Art”, and we hope that the liminal world of “Art” can be an effective medium to transmit the sometimes counterintuitive results of empirical “Science” to a broader audience, also generating a dialogue between the two. We will describe the scientific concepts that currently inspire us, the process by which we convert quantum principles into movements, and the challenges of distilling this into a theatrical setting.
What is Quantum Physics? Dancers Explain, NIST
Sam Mitchel Dance: Dunamis Novem